Respiratory Health Abstract: Diet and nutrition may be important modifiable risk factors for the development,
progression and management of obstructive lung diseases such as asthma and chronic
obstructive pulmonary disease (COPD). This review examines the relationship between
dietary patterns, nutrient intake and weight status in obstructive lung diseases, at different life stages, from in-utero influences through childhood and into adulthood. In vitro and animal studies suggest important roles for various nutrients, some of which are supported by epidemiological studies. However, few well-designed human intervention trials are available to definitively assess the efficacy of different approaches to nutritional management of respiratory diseases. Evidence for the impact of higher intakes of fruit and vegetables is amongst the strongest, yet other dietary nutrients and dietary patterns require evidence from human clinical studies before conclusions can be made about their effectiveness.
Keywords: respiratory disease; asthma; COPD; dietary patterns; antioxidants; vitamin C;
vitamin E; flavonoids; vitamin D; obesity; adipokines; undernutrition
Table of Contents
1. Introduction: Respiratory Health
Diet and nutrition are increasingly becoming recognized as modifiable contributors to chronic disease development and progression. Considerable evidence has emerged indicating the importance of dietary intake in obstructive lung diseases such as asthma and chronic obstructive pulmonary disease (COPD) in both early life and disease development [1,2] and management of disease progression [3,4]. These respiratory diseases are characterized by airway and systemic inflammation, airflow obstruction, deficits in lung function and significant morbidity and mortality, as well as being costly economic burdens [5,6]. Pharmacological management remains the mainstay for treatment of respiratory diseases, and while treatment options are advancing, dietary intake modification could be an important adjuvant to disease management and an important consideration for disease prevention. Dietary patterns as well as intake of individual nutrients have been evaluated in observational and experimental studies throughout life stages and disease stages to elucidate their role in respiratory diseases. This review concentrates on evidence regarding the role of dietary patterns, individual nutrients, weight status and adipokines in asthma and COPD.
2. Dietary Intake And Respiratory Diseases
2.1. Dietary Patterns: Respiratory Health
Various dietary patterns have been linked to the risk of respiratory disease [7]. The Mediterranean diet has been found to have protective effects for allergic respiratory diseases in epidemiological studies [8]. This dietary pattern consists of a high intake of minimally processed plant foods, namely; fruit, vegetables, breads, cereals, beans, nuts and seeds, low to moderate intake of dairy foods, fish, poultry and wine and low intake of red meat. High intakes of olive oil result in a dietary composition that is low in saturated fat though still moderate in total fat. In children, several studies showed that adherence to the Mediterranean diet is inversely associated with atopy and has a protective effect on atopy, wheezing and asthma symptoms [9–11]. The Mediterranean diet may also be important for maternal diet, as a study in Spain found that a high Mediterranean diet score during pregnancy was protective for persistent wheeze and atopic wheeze in children at 6.5 years of age [12]. Though one cross-sectional study in Japan reported a strong association between the adherence to the Mediterranean diet and asthma control [13], there is less evidence available to support this dietary pattern in adults. The “western” dietary pattern, prevalent in developed countries, is characterised by high consumption of refined grains, cured and red meats, desserts and sweets, french fries, and high-fat dairy products [2,14]. This pattern of intake has been associated with increased risk of asthma in children [15,16]. Furthermore, in children, increased intake of fast food such as hamburgers and related eating behaviours, for example salty snack eating and frequent take away consumption, are correlated with the presence of asthma, wheezing and airway hyperresponsiveness (AHR) [17,18]. In adults, a western diet has been shown to be positively associated with increased frequency of asthma exacerbation [19], but not related to asthma risk. In addition, an acute challenge with a high fat fast food meal has been shown to worsen airway inflammation [20]. While this dietary pattern appears to be deleterious in children and adults with asthma, studies examining the effect of this dietary pattern in maternal diets have found no relationship with a consumption of a “western” style diet in pregnancy and risk of asthma in offspring [21]. Cross-sectional studies have also found that the “western” diet is associated with an increased risk of COPD [2]. In summary the Mediterranean diet appears to be protective in children, though there is less evidence for benefits in the maternal diet and in adults. There is evidence to suggest that a “western” style dietary pattern increases risk of asthma in children, has worse outcomes for adults with asthma and is related to COPD risk.
2.2. Fruit And Vegetables: Respiratory Health
Fruit and vegetable intake has been investigated for potential benefits in association with respiratory conditions due to their nutrient profile consisting of antioxidants, vitamins, minerals, fibre and phytochemicals. The mechanisms by which the nutrients in fruit and vegetables exert beneficial effects in respiratory conditions are discussed in the sections below. Epidemiological evidence reviewed by Saadeh et al. [7] showed that fruit intake was associated with a low prevalence of wheezing and that cooked green vegetable intake was associated with a low prevalence of wheezing and asthma in school children aged 8–12 years old. Furthermore low vegetable intake in children was related to current asthma [7]. In adults, Grieger et al. [22] discusses the heterogeneous nature of the data describing fruit and vegetable intake and lung function, with one study showing no effect on lung function of higher fruit and vegetable intake over 10 years [23], yet in another study, increased fruit intake over 2 years was associated with increased FEV1 [23], while another study showed that a large decrease in fruit intake over 7 years was associated with decreased FEV1 [24]. We recently conducted an intervention in adults with asthma and found that subjects who consumed a high fruit and vegetable diet for 3 months, had a decreased risk of asthma exacerbation, compared to subjects who consumed a low fruit and vegetable diet [25]. A recent meta-analysis of adults and children, which analysed 12 cohorts, 4 population-based case-control studies, and 26 cross-sectional studies provides important new evidence showing that a high intake of fruit and vegetables reduces the risk of childhood wheezing, and that fruit and vegetable intake is negatively associated with asthma risk in adults and children [26]. While some studies of maternal diet have found no relationship with fruit and vegetable intake and asthma in children [27], other studies have found that increased fruit and vegetable intake were related to a decreased risk of asthma in children [21,28]. Increased fruit and vegetable intake may be protective against COPD development, with consumption of a “prudent” diet including increased fruit and vegetables being protective against lung function decline [3]. Two randomized controlled trials (RCT’s) manipulating fruit and vegetable intake have been conducted in COPD. A 12 week study showed no effect of a high fruit and vegetable intake on FEV1, systemic inflammation or airway oxidative stress [29]. However, a 3-year study in 120 COPD patients revealed an improvement in lung function in the high fruit and vegetable group compared to the control group [30], suggesting that longer term intervention is needed to provide a therapeutic effect. There is considerable evidence to suggest that a high intake of fruit and vegetables is favourable for all life stages of asthma and evidence is emerging which suggests the same in COPD.
2.3. Omega-3 Fatty Acids And Fish: Respiratory Health
Omega-3 polyunsaturated fatty acids (PUFA) from marine sources and supplements have been shown to be anti-inflammatory through several cellular mechanisms including their incorporation into cellular membranes and resulting altered synthesis of eicosanoids [31]. Experimental studies have shown that long chain omega-3 PUFA’s decrease inflammatory cell production of pro-inflammatory prostaglandin (PG) E2, leukotriene (LT) B4 [32] and activity of nuclear factor-kappaB (NF-?B), a potent inflammatory transcription factor [33]. Long chain omega-3 PUFA’s also down regulate pro-inflammatory cell cytokine production (interleukin-1? (IL-1?), tumor necrosis factor-? (TNF-?)) by monocytes and macrophages, decrease expression of cellular adhesion molecules on monocytes and endothelial cells and reduce production of ROS in neutrophils [34]. Saddeh et al. [7] reported that the evidence describing the relationship between omega-3 PUFA’s or fish consumption and respiratory conditions in childhood is contradictory. Some observational studies show that intake of oily fish is negatively associated with AHR and asthma [35,36]. However, evidence from Japan suggests that frequency of fish consumption is positively related to asthma risk [37] and in Saudi Arabia fish intake was not related to the presence of asthma or wheezing at all [18]. Similarly in adults, the data is heterogeneous, with omega-3 PUFAs or fish being associated with improved lung function [38] and decreased risk of asthma [39], AHR [35] and wheeze [36] in some, but not all studies [40]. Maternal dietary intake of oily fish was found to be protective of asthma in children 5 years of age if born to mothers with asthma [41] and a recent systematic review of omega-3 fatty acid supplementation studies in women during pregnancy found that the risk of asthma development in children was reduced [42]. The data examining the possible benefits of dietary omega-3 fatty acid supplementation in asthma are heterogeneous and as summarized by a 2002 Cochrane review [43], to date there is insufficient evidence to recommend omega-3 PUFA supplementation in asthma. Omega-3 PUFA may have positive effects in COPD, as higher levels of DHA in serum were found to decrease the risk of developing COPD [44]. Experimental studies in humans with COPD including supplementation with omega-3 found lower levels of TNF-? [45] and improved rehabilitation outcomes [46], though no improvements were seen in FEV1. Several studies using omega-3 PUFA supplementation in COPD are currently underway and will provide important new information to inform the field [47–49]. Consumption of oily fish or supplementation with omega-3 PUFA’s may have positive effects in asthma and COPD, though strong evidence to support the experimental and epidemiological data is not yet available.
3. Nutrients And Respiratory Disease
3.1. Antioxidants And Oxidative Stress
Dietary antioxidants are an important dietary factor in protecting against the damaging effects of oxidative stress in the airways, a characteristic of respiratory diseases [50]. Oxidative stress caused by reactive oxygen species (ROS), is generated in the lungs due to various exposures, such as air pollution, airborne irritants and typical airway inflammatory cell responses [51]. Also, increased levels of ROS generate further inflammation in the airways via activation of NF-?B and gene expression of pro-inflammatory mediators [52]. Antioxidants including vitamin C, vitamin E, flavonoids and carotenoids are abundantly present in fruits and vegetables, as well as nuts, vegetable oils, cocoa, red wine and green tea. Dietary antioxidants may have beneficial effects on respiratory health, from influences of the maternal diet on the fetus, and intake in children through to adults and pregnant women with asthma and adults with COPD. ?-tocopherol is a form of vitamin E, which helps maintain integrity of membrane fatty acids, by inhibiting lipid peroxidation [22]. Carotenoids are plant pigments and include; ?- and ?-carotene, lycopene, lutein and ?-cryptoxanthin. This group of fat soluble antioxidants have been shown to benefit respiratory health due to their ability to scavenge ROS and reduce oxidative stress [22]. The antioxidant lycopene, present predominantly in tomatoes, may be beneficial in respiratory conditions, indeed lycopene intake has been positively correlated with FEV1 in both asthma and COPD [53] and an intervention study in asthma showed that lycopene supplementation could suppress neutrophilic airway inflammation [54]. Antioxidants may also be important in asthma during pregnancy, as while oxidative stress commonly increases during normal pregnancies, in women with asthma oxidative stress is heightened [55]. During pregnancy there is a compensatory increase in circulating and placental antioxidants in asthma versus women without asthma, to protect the foetus against damaging effects of oxidative stress [55,56]. Improving antioxidant intake in pregnant women with asthma may be beneficial as poor fetal growth outcomes are associated with low levels of circulating antioxidants and dietary antioxidants are the first defense mechanism against ROS [22]. Maternal intake of vitamin E, vitamin D, milk, cheese and calcium during pregnancy are negatively associated, while vitamin C is positively associated, with wheezing in early childhood [57,58]. Antioxidants including lycopene appear to have positive influences in respiratory conditions, further detail is provided below on evidence for vitamin C, vitamin E and flavonoids and their role in the maternal diet, diets of children and adults with asthma and adults with COPD.
3.2. Vitamin C: Respiratory Health
Vitamin C has been enthusiastically investigated for benefits in asthma and links to asthma prevention. In vitro data from endothelial cell lines showed that vitamin C could inhibit NF-?B activation by IL-1, TNF-? and block production of IL-8 via mechanisms not dependent on the antioxidant activity of vitamin C [59]. Anti-inflammatory and anti-asthmatic effects of vitamin C supplementation in vivo, have been shown through allergic mouse models of asthma. Jeong et al. [60] reported decreased AHR to methacholine and inflammatory cell infiltration of perivascular and peribronchiolar spaces when vitamin C was supplemented during allergen challenge. While Chang et al. [61] found that high dose Vitamin C supplementation in allergen challenged mice decreased eosinophils in BALF and increased the ratio of Th1/Th2 cytokine production shifting the inflammatory pattern to Th1 dominant. Observational studies in children showed consumption of fruit, a rich source of vitamin C, was related to reduced wheezing [62] and vitamin C intake was negatively associated with wheezing [63], while another study reported no relationship between vitamin C intake and lung function [64]. Grieger et al. [22] also reported conflicting evidence for effects of vitamin C intake in adults, with epidemiological studies showing a positive association between vitamin C intake and lung function in some [65], but not all studies [23,66]. Despite the observational data linking vitamin C to lung health, supplementation with vitamin C has not been shown to reduce the risk of asthma [66] which may be related to the interdependence of nutrients found in foods, resulting in lack of efficacy when supplementing with isolated nutrients. Evidence from experimental and observational studies suggests that Vitamin C might be important in COPD pathogenesis and management. Koike et al. [67] reported that in knock out mice unable to synthesize vitamin C, vitamin C supplementation was able to prevent smoke induced emphysema and also to restore damaged lung tissue and decrease oxidative stress caused by smoke induced emphysema. A case control study in Taiwan reported that subjects with COPD had lower dietary intake and lower serum levels of vitamin C than healthy controls [68]. Indeed an epidemiological study in the United Kingdom of over 7000 adults aged 45–74 years found that increased plasma vitamin C concentration was associated with a decreased risk of obstructive airways disease, suggestive of a protective effect [69]. Thus, in summary, while observational data has suggested that vitamin C is important for lung health, intervention trials showing efficacy are lacking and it appears that supplementation with vitamin C-rich whole foods, such
as fruit and vegetables may be more effective.
3.3. Vitamin E: Respiratory Health
The vitamin E family comprises of 4 tocopherols and 4 tocotrienols, with the most plentiful in the diet or in tissues being ?-tocopherol and ?-tocopherol [70]. Vitamin E works synergistically with vitamin C, as following neutralisation of ROS, oxidised vitamin E isoforms can be processed back into their reduced form by vitamin C [71]. Abdala-Valencia et al. [72] discuss the evidence for the roles of ?-tocopherol and ?-tocopherol in allergic lung inflammation in mechanistic animal studies and clinical trials. Supplementation of mice with ?-tocopherol reduced allergic airway inflammation and AHR [73], while ?-tocopherol was pro-inflammatory and augmented AHR, negating the positive effects of ?-tocopherol [74]. Other animal studies report that ?-tocopherol may assist in resolving inflammation caused by ozone exposure and endotoxin induced neutrophilic airway inflammation, owing to its ability to oxidize reactive nitrogen species [75,76]. A study in humans showed that both ? and ?-tocopherol may be effective in decreasing LPS induced neutrophilic inflammation [77]. The conflicting results from these supplementation studies are likely to be influenced by baseline tissue levels of vitamin E [72], with ?-tocopherol supplementation leading to improved lung function and wheeze in Europe, where ?-tocopherol levels are low [78–80], but not in the US, where ?-tocopherol intake is high due to soybean oil consumption [81–83]. As a result, meta-analysis of vitamin E effects on asthma outcomes is equivocal; it is likely that supplementation with physiological concentrations of ?-tocopherol in the context of a background diet low in ?-tocopherol, may be most beneficial in asthma and further research testing this hypothesis is required. In COPD, serum levels of vitamin E have been shown to be decreased during exacerbation, which suggests increased intake may be helpful to improve vitamin E concentrations [84]. Vitamin E has been shown to reduce biomarkers of oxidative stress in adults with COPD in one RCT [85], but not another [86]. In the Women’s Health Study (n = 38,597), the risk of developing chronic lung disease over a 10 year supplementation period was reduced by 10% in women using vitamin E supplements (600 IU on alternate days) [87]. Dietary intake of vitamin E lower than recommended dietary intakes has been reported in pregnant women with a family history of allergic disease [88] and recent work in animal models has highlighted ?-tocopherol may be important for allergic mothers in pregnancy. Allergic female mice were supplemented with ?-tocopherol prior to mating and following allergen challenge the offspring showed reduced response to allergen challenge with decreased eosinophils in BALF [89]. The offspring also showed reduced development of lung dendritic cells, necessary for producing allergic responses. Evidence from observational studies also suggests that reduced maternal dietary intake of vitamin E is related to an increased risk of childhood asthma and wheeze [90–92] and increased in vitro proliferative responses in cord blood mononuclear cells (CBMC) [93]. A mechanistic study by Wassall et al. [94] examined the effect of ?-tocopherol and vitamin C on CBMC and maternal peripheral blood mononuclear cells (PBMC). ?-tocopherol was mostly anti-inflammatory, although increased proliferation and increased TGF-? were seen with some allergens. However, the addition of vitamin C to the system had inflammatory actions, with increased production of pro-inflammatory cytokines, combined with reduced production of IL-10 and TGF-?. This study by Wassall et al. [94] demonstrates that supplementation with these antioxidants does modulate immune responses in pregnancy, however several of the results are unexpected, highlighting the complex nature of the relationships between dietary nutrients and disease. In asthma the experimental data for vitamin E are compelling, yet supplementation benefits are not well described. In COPD there is currently not enough evidence to make conclusions about vitamin E supplementation.
3.4. Flavonoids: Respiratory Health
Flavonoids are potent antioxidants and have anti-inflammatory as well as anti-allergic actions due in part, to their ability to neutralise ROS [95]. There are 6 classes of flavonoids including flavones, flavonols, flavanones, isoflavones and flavanols [96], which are widely distributed throughout the diet and found in fruit, vegetables, nuts, seeds, stems, flowers, roots, bark, dark chocolate, tea, wine and coffee [96]. Tanaka et al. [95] present the evidence for the benefits of dietary flavonoids in asthma development and progression. In addition to reducing oxidative stress, in vitro experiments have found that many individual flavonoids have inhibitory effects on IgE mediated immune responses such as histamine secretion by mast cells, shift in cytokine production from Th-2 to Th-1 production and decreased NF-?B activation and inhibition of TNF-? [97–100]. Experimental studies of flavonoids in animal models of allergic asthma have shown reduced airway and peripheral blood inflammation, decreased bronchoconstriction and AHR and lower eosinophils in BALF, blood and lung tissue [101–104]. In humans, evidence from a case control study in adults showed that apple and red wine consumption, rich sources of flavonoids, was associated with reduced asthma prevalence and severity [66]. However a follow-up study investigating intake of 3 subclasses of flavonoids did not find any associations with asthma prevalence or severity [105]. There are a limited number of experimental studies using flavonoid supplements in humans with asthma. Three RCT’s in adults with asthma using a product called pycnogenol, which contains a mixture of bioflavonoids, reported benefits including increased lung function, decreased symptoms and reduced need for rescue inhalers [106]. There is a paucity of evidence for the effects of flavonoids in the maternal diet and respiratory outcomes in children. One study which found a positive association of maternal apple intake and asthma in children at 5 years, suggests that the flavonoid content of apples may be responsible for the beneficial relationship [107]. Evidence for the effects of flavonoids in respiratory conditions is emerging and promising. Though like vitamin C, it may be difficult to disentangle the effects of flavonoids from other nutrients in flavonoid-rich foods. Supplementation of individual flavonoids in experimental animal studies has provided evidence to suggest that intervention trials in humans may be warranted.
3.5. Vitamin D: Respiratory Health
Epidemiological studies show promising associations between vitamin D and lung health; however the mechanisms responsible for these effects are poorly understood. Vitamin D can be obtained from dietary sources or supplementation; however sun exposure is the main contributor to vitamin D levels [108]. While vitamin D has beneficial effects independent of UV exposure [109], it can be difficult to separate this potential confounder from direct effects of vitamin D on lung health [110]. The review by Foong and Zosky [111] presents the current evidence for the role of vitamin D deficiency in disease onset, progression and exacerbation in respiratory infections, asthma and COPD. Respiratory infections contribute to disease progression and exacerbation in both COPD and asthma. Vitamin D appears to have a protective role against the susceptibility to and severity of these infections [111], as active vitamin D (1,25 (OH)2D) modifies production of antimicrobial cathelicidins and defensins that kill bacteria and induce wound repair [112]. Activated vitamin D also decreases the expression of rhinovirus receptors in endothelial cell cultures and PBMC’s [113]. In vitro studies also support the link between vitamin D and airway remodelling as active vitamin D inhibits airway smooth muscle (ASM) cell proliferation [114] and deficiency impairs normal lung development [115]. Furthermore, animal models suggest that vitamin D can inhibit Th1 and Th2 cell cytokine production [116]. Epidemiological evidence links low levels of vitamin D with wheeze and respiratory infections, though evidence for the link with asthma onset is weak and inconsistent [111]. In children, low circulating vitamin D was related to lower lung function, increased corticosteroid use and exacerbation frequency [117]. Also in children with steroid resistant asthma, low vitamin D was related to increased ASM thickness [117]. Other observational studies report that in children, low levels of vitamin D are associated with asthma exacerbation [118]. Several observational studies support the role of vitamin D for protection against respiratory conditions in children. Zosky et al. [119] found that vitamin D deficiency at 18 weeks gestation was associated with lower lung function and current wheeze in children 6 years of age and an increased risk of asthma in boys. The role for vitamin D in enhancing steroid responsiveness suggested by observational studies [120] is supported by mechanistic studies [121], and in concert with the actions of vitamin D in infection, may explain the effect of vitamin D in reducing asthma exacerbations [111]. Only one intervention trial has been conducted using vitamin D in adults with asthma, which found that rate of first exacerbation was reduced in subjects who demonstrated an increase in circulating vitamin D3 following supplementation [122]. Data for the role of vitamin D in COPD onset is limited, though several cross-sectional studies have reported an association between low vitamin D levels, or deficiency, with COPD incidence [123]. Blood vitamin D levels have also been correlated with lung function in COPD patients [124,125]. Experimental data suggest that vitamin D may be important in COPD for its effect on normal lung growth and development, though human data to support this is not available. It is possible that COPD onset may also be impacted by cellular responses to cigarette smoke exposure which inhibits the protective immunomodulatory effects of vitamin D [126]. There is research suggesting a genetic link between vitamin D and COPD pathogenesis. In an observational study single nucleotide polymorphisms in the vitamin D binding protein (VDBP) predicted vitamin D levels in COPD patients and were found to be a risk factor for COPD [123]. The VDBP is also involved in macrophage activation as high levels of airway VDBP are related to increased macrophage activation, also high levels of serum VDBP were found to be related to lower lung function [127]. COPD progression may also be affected by vitamin D status through absence of the vitamin D receptor and parenchyma degradation [128]. COPD exacerbations are generally caused by viral or bacterial lung infections, and though vitamin D has a positive role in reducing infection, there is no evidence to support that vitamin D is associated with ameliorating exacerbations in COPD patients [129]. The extra-skeletal effects of vitamin D are well documented in both asthma and COPD, and deficiency is associated with negative respiratory and immune outcomes. At this stage however, more evidence from supplementation interventions is needed before widespread adoption of supplementation can be recommended.
3.6. Minerals: Respiratory Health
Some minerals have also been found to be protective in respiratory conditions. In children, increased intake of magnesium, calcium and potassium is inversely related to asthma prevalence [7]. While several observational and experimental trials have been performed with conflicting results [130], a randomised controlled trial concluded that a low sodium diet had no therapeutic benefit for bronchial reactivity in adults with asthma [131]. Dietary magnesium may have beneficial bronchodilator effects in asthma [132]. Low dietary magnesium intake has been associated with negative effects on bronchial smooth muscle in severe asthma [133] and with lower lung function in children [134]. However further evidence of positive therapeutic effects are required before its importance in asthma and recommendations can be determined [135]. Dietary intake of selenium has been shown to be lower in asthmatics compared to non-asthmatics [136] and maternal plasma selenium levels were reported to be inversely associated with risk of asthma in children [137]. However case control studies in children have not found a relationship with selenium levels or intake with asthma related outcomes [18,138]. Furthermore, results from a large well designed RCT in adults with asthma showed no positive benefit of selenium supplementation [139]. Investigation of minerals in cord blood imply the importance of adequate intake during pregnancy, as levels of cord blood selenium were negatively associated with persistent wheeze, and levels of iron were negatively associated with later onset wheeze in children [140]. Studies on dietary intake of minerals and associations with COPD are sparse. A small study in Sweden found that in older subjects with severe COPD, intakes of folic acid and selenium were below recommended levels, and although intake of calcium was adequate, serum calcium levels were low, likely related to their vitamin D status as intake was lower than recommended [141]. Mineral intake may be important in respiratory diseases, yet evidence for supplementation is weak. It is likely that adequate intake of these nutrients in a whole diet approach is sufficient.
4. Obesity, Adipokines And Respiratory Disease
Overnutrition and resulting obesity are clearly linked with asthma, though the mechanisms involved are still under investigation. The review by Periyalil et al. [142] describes how immunometabolismadipose tissue derived immunological changes causing metabolic effects [143] contributes to the link between asthma and obesity. In the obese state dietary intake of lipids leads to increased circulating free fatty acids [144], which activate immune responses, such as activation of TLR4, leading to increased inflammation, both systemically and in the airways [20]. Adipose tissue also secretes adipokines and asthmatic subjects have higher concentrations of circulating leptin than healthy controls [14] which are further increased in females, though leptin is associated with BMI in both males and females [145]. Leptin receptors are present in the bronchial and alveolar epithelial cells and leptin has been shown to induce activation of alveolar macrophages [146] and have indirect effects on neutrophils [147]. Also leptin promotes Th1 proliferation inducing increased activation of neutrophils by TNF-? [148]. In vitro, leptin also activates alveolar macrophages taken from obese asthmatics, which induces airway inflammation through production of pro-inflammatory cytokines [149]. However, a causal role for leptin in the obese asthma relationship is yet to be established. Adiponectin, an anti-inflammatory adipokine, has beneficial effects in animal models of asthma [150], however, positive associations in human studies have only been seen in women [151]. In obesity, macrophage and mast cell infiltration into adipose tissue is upregulated [142]. Neutrophils also appear to dominate airway inflammation in the obese asthma phenotype [152], particularly in females [153], which may explain why inhaled corticosteroids are less effective in achieving control in obese asthma [154]. While the mechanisms are yet to be understood, a recent review reports that obesity in pregnancy is associated with higher odds of asthma in children, with increased risk as maternal BMI increases [155].
COPD is characterised not only by pulmonary deficits but also by chronic systemic inflammation and co-morbidities which may develop in response to the metabolic dysregulation that occurs with excess adipose tissue [156]. A recent meta-analysis of leptin levels in COPD reported a correlation with body mass index (BMI) and fat mass percent in stable COPD though absolute levels were not different to healthy controls [157]. During exacerbation, leptin levels increased and were positively associated with circulating TNF-? [157]. Bianco et al. [158] describes the role of adiponectin and its effect on inflammation in COPD. Adiponectin has anti-inflammatory effects and is present in high concentrations in serum of healthy subjects [159]. Adiponectin exists in several isoforms, which have varied biological effects [160] and interact with two receptors present in the lungs (AdipoR1 and AdipoR2) that have opposing effects on inflammation [161]. Single nucleotide polymorphisms in the gene encoding adiponectin are associated with cardiovascular disease, obesity and the metabolic syndrome [162]. The role of adiponectin in COPD however is not well understood. In COPD, serum adiponectin is increased and directly relates to disease severity and lung function decline [163]. There is an alteration in the oligomerisation of adiponectin in COPD resulting in increased concentrations of the anti-inflammatory higher-molecular weight isoform [164], and the expression of adiponectin receptors in the lung is also altered in comparison to healthy subjects [165]. Animal models have shown anti-inflammatory effects of adiponectin in the lung through the increased expression of TNF-? in alveolar macrophages in adiponectin deficient mice [166]. Further mechanistic studies have also shown the anti-inflammatory potential of adiponectin by reducing the effects of TNF-?, IL-1? and NF-?B and increasing expression of IL-10 through interaction with AdipoR1 [161]. However under certain conditions in cell lines and animal models adiponectin has been shown to have pro-inflammatory effects [167,168]. As both detrimental and protective effects have been seen, the complex modulation of adiponectin isoforms and receptors in COPD requires further exploration. Obesity, the resulting systemic inflammation and alterations in adipokines have significant negative effects in both asthma and COPD. While work examining the mechanisms of effect is extensive, evidence for interventions to improve the course of disease are limited to weight loss interventions in asthma at this stage.
5. Undernutrition And Respiratory Disease
Though underweight has not been well studied in asthma, an observational study in Japan reported that subjects with asthma who were underweight had poorer asthma control than their normal weight counterparts [169]. While there is widespread acknowledgement that malnutrition in pregnant women adversely effects of the lung development of the fetus [170], a recent review reported that the offspring of mothers who were underweight did not have an increased risk of asthma. Amongst the obstructive lung diseases, undernutrition is most commonly recognized as a feature of COPD. Itoh et al. [171] present a review on undernutrition in COPD and the evidence for nutritional therapy in management of the disease. Weight loss, low body weight and muscle wasting are common in COPD patients with advanced disease and are associated with reduced survival time and an increased risk of exacerbation [172]. The causes of undernutrition in COPD are multifactorial and include reduced energy intake due to decreased appetite, depression, lower physical activity and dyspnoea while eating [173]. In addition, resting energy expenditure is increased in COPD, likely due to higher energy demands from increased work of breathing [174]. Also, systemic inflammation which is a hallmark of COPD, may influence energy intake and expenditure [175]. Cigarette smoke may also have deleterious effects on body composition in addition to the systemic effects of COPD. Smoking causes muscle fibre atrophy and decreased muscle oxidative capacity shown in cohorts of non-COPD smokers [176,177] and in animal models of chronic smoke exposure [178,179]. The mechanisms underlying muscle wasting in COPD are complex and multifaceted [180]. Increased protein degradation occurs in the whole body, though it is enhanced in the diaphragm [181]. Protein synthesis pathways are altered, indeed insulin like growth factor-1 (IGF-1) which is essential for muscle synthesis is decreased in cachectic COPD patients [182] and is lower in COPD patients during acute exacerbation, compared to healthy controls [183]. Increased oxidative stress, due to increased mitochondrial ROS production, occurs both systemically and in muscle tissue in cachectic COPD patients and is negatively associated with fat free mass (FFM) and muscle strength in COPD patients [184]. Furthermore myostatin induces muscle atrophy by inhibiting proliferation of myoblasts and mRNA expression of myostain is increased in cachectic COPD patients and is related to muscle mass [185]. Systemic inflammatory mediators such as TNF-? and NF-?B are also implicated in COPD muscle atrophy [186,187]. Nutritional supplementation therapy in undernourished COPD patients has been shown to induce weight gain, increase fat free mass, increase grip strength and exercise tolerance as well as improve quality of life [188]. Further studies point out the importance of not only high energy content, but also macronutrient composition of the nutritional supplement and inclusion of low intensity respiratory rehabilitation exercise [189,190]. Other dietary nutrients have been investigated for the benefits in COPD. Creatinine, found in meat and fish, did not have additive effects to rehabilitation, while sulforaphane, found in broccoli and wasabi, and curcumin, the pigment in turmeric, may have beneficial antioxidant properties [191–193]. Branched chain amino acid supplementation in COPD is associated with positive results including increases in whole body protein synthesis, body weight, fat free mass and arterial blood oxygen levels [194,195]. Undernutrition is not a significant problem in asthma, though is a major debilitating feature of COPD. There is promising evidence that nutritional supplementation in COPD is important and can help to alleviate some of the adverse effects of the disease, particularly muscle wasting and weight loss.
6. Conclusions: Respiratory Health
Dietary intake appears to be important in both the development and management of respiratory diseases, shown through epidemiological and cross-sectional studies and supported by mechanistic studies in animal models. Although more evidence is needed from intervention studies in humans, there is a clear link for some nutrients and dietary patterns. The dietary patterns associated with benefits in respiratory diseases include high fruit and vegetable intake, Mediterranean style diet, fish and omega-3 intake, while fast food intake and westernized dietary patterns have adverse associations. Figure 1 shows a diagrammatic representation of the relationships of nutrition and obstructive lung diseases.
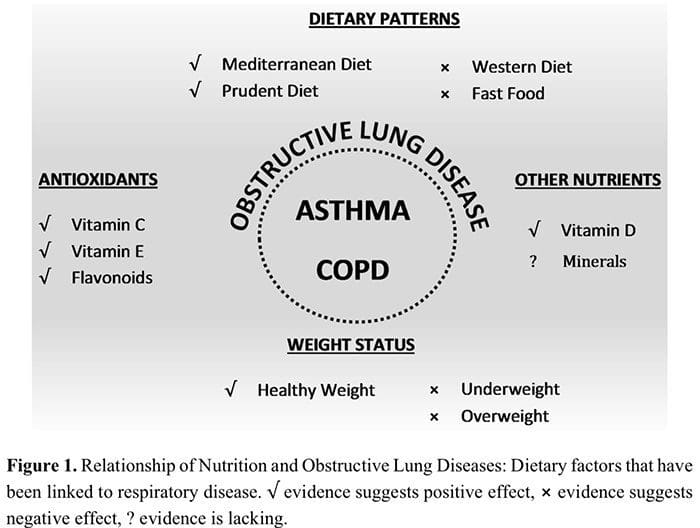
Though antioxidants are associated with positive effects on inflammation, clinical outcomes and respiratory disease prevention, intervention studies of individual antioxidants do not indicate widespread adoption of supplementation [196]. Differences in results from individual studies including whole foods such as fruit and vegetables and fish could be influenced by the nutritional profile owing to the region it was grown or produced. In considering studies using single nutrients it is also important to acknowledge that nutrients in the diet are consumed as whole foods that contain other micronutrients, fibre and compounds with both known and unknown anti and pro-inflammatory potential. Furthermore investigations of single nutrients should ideally control for other antioxidants and dietary sources of pro-inflammatory nutrients. While this limitation is common, it is a significant challenge to control for dietary intake of other nutrients in clinical trials. A whole foods approach to nutrient supplementation—for example, increasing intake of fruit and vegetables, has the benefit of increasing intake of multiple nutrients, including vitamin C, vitamin E, carotenoids and flavonoids and shows more promise in respiratory diseases in terms of reducing risk of COPD [3] and incidence of asthma exacerbations [25].
The evidence for mechanisms of vitamin D in lung development and immune function are yet to be fully established. It appears that vitamin D is important in respiratory diseases and infections, however the temporal role of vitamin D deficiency in disease onset, pathogenesis and exacerbations and whether supplementation is indicated is yet to be clarified.
Overnutrition in respiratory disease is clearly associated with adverse effects, highlighted by detrimental effects induced by immunometabolism. Further understanding of the relationship between mediators of immunometabolism and respiratory diseases and their mechanisms may provide therapeutic options. Undernutrition still poses risk in some respiratory conditions. Appropriate nutritional supplementation in advanced COPD is indicated, and several nutrients appear to be beneficial in COPD development and exacerbation.
The field of nutrition and respiratory disease continues to develop and expand, though further work is required in the form of randomized controlled dietary manipulation studies using whole foods to enable provision of evidence based recommendations for managing respiratory conditions.
Bronwyn S. Berthon and Lisa G. Wood *
Centre for Asthma and Respiratory Diseases, Level 2, Hunter Medical Research Institute,
University of Newcastle, Lot 1 Kookaburra Circuit, New Lambton Heights, NSW 2305, Australia;
E-Mail: bronwyn.berthon@newcastle.edu.au
* Author to whom correspondence should be addressed; E-Mail: lisa.wood@newcastle.edu.au;
Tel.: +61-2-4042-0147; Fax: +61-2-4042-0046.
Author Contributions
Bronwyn Berthon and Lisa Wood contributed to the study concept and design and were both involved in the preparation and completion of the manuscript.
Conflicts of Interest
The authors declare no conflicts of interest.
© 2015 by the authors; licensee MDPI, Basel, Switzerland. This article is an open access article
distributed under the terms and conditions of the Creative Commons Attribution license
(creativecommons.org/licenses/by/4.0/).
1. Nurmatov, U.; Devereux, G.; Sheikh, A. Nutrients and foods for the primary prevention of asthma<br>
and allergy: Systematic review and meta-analysis. J. Allergy Clin. Immunol. 2011, 127,<br>
724–733.e30.<br>
2. Varraso, R.; Fung, T.T.; Barr, R.G.; Hu, F.B.; Willett, W.; Camargo, C.A.J. Prospective study of<br>
dietary patterns and chronic obstructive pulmonary disease among US women. Am. J. Clin. Nutr.<br>
2007, 86, 488–495.<br>
3. Shaheen, S.O.; Jameson, K.A.; Syddall, H.E.; Aihie Sayer, A.; Dennison, E.M.; Cooper, C.;<br>
Robinson, S.M.; Hertfordshire Cohort Study Group. The relationship of dietary patterns with adult<br>
lung function and COPD. Eur. Respir. J. 2010, 36, 277–284.<br>
4. Scott, H.A.; Jensen, M.E.; Wood, L.G. Dietary interventions in asthma. Curr. Pharm. Des. 2014,<br>
20, 1003–1010.<br>
5. Global Initiative for Asthma (GINA). Global Strategy for Asthma Management and Prevention<br>
2012 (update). Available online: www.ginasthma.org (accessed on 30 July 2013).<br>
6. Global Initiative for Chronic Obstructive Lung Disease (GOLD). Global Strategy for the<br>
Diagnosis, Management and Prevention of COPD. Available online: www.goldcopd.org/<br>
(accessed on 3 December 2014).<br>
7. Saadeh, D.; Salameh, P.; Baldi, I.; Raherison, C. Diet and allergic diseases among population aged<br>
0 to 18 years: Myth or reality? Nutrients 2013, 5, 3399–3423.<br>
8. Willett, W.C.; Sacks, F.; Trichopoulou, A.; Drescher, G.; Ferro-Luzzi, A.; Helsing, E.;<br>
Trichopoulos, D. Mediterranean diet pyramid: A cultural model for healthy eating. Am. J. Clin.<br>
Nutr. 1995, 61, 1402S–1406S.<br>
9. Arvaniti, F.; Priftis, K.N.; Papadimitriou, A.; Papadopoulos, M.; Roma, E.; Kapsokefalou, M.;<br>
Anthracopoulos, M.B.; Panagiotakos, D.B. Adherence to the Mediterranean type of diet is associated<br>
with lower prevalence of asthma symptoms, among 10–12 years old children: The PANACEA<br>
study. Pediatr. Allergy Immunol. 2011, 22, 283–289.<br>
10. Chatzi, L.; Kogevinas, M. Prenatal and childhood Mediterranean diet and the development of<br>
asthma and allergies in children. Public Health Nutr. 2009, 12, 1629–1634.<br>
11. De Batlle, J.; Garcia-Aymerich, J.; Barraza-Villarreal, A.; Antó, J.M.; Romieu, I. Mediterranean<br>
diet is associated with reduced asthma and rhinitis in Mexican children. Allergy 2008, 63,<br>
1310–1316.<br>
12. Chatzi, L.; Torrent, M.; Romieu, I.; Garcia-Esteban, R.; Ferrer, C.; Vioque, J.; Kogevinas, M.;<br>
Sunyer, J. Mediterranean diet in pregnancy is protective for wheeze and atopy in childhood. Thorax<br>
2008, 63, 507–513.<br>
13. Barros, R.; Moreira, A.; Fonseca, J.; de Oliveira, J.F.; Delgado, L.; Castel-Branco, M.G.; Haahtela,<br>
T.; Lopes, C.; Moreira, P. Adherence to the Mediterranean diet and fresh fruit intake are associated<br>
with improved asthma control. Allergy 2008, 63, 917–923.<br>
14. Wood, L.G.; Gibson, P.G. Dietary factors lead to innate immune activation in asthma. Pharmacol.<br>
Ther. 2009, 123, 37–53.<br>
15. Carey, O.J.; Cookson, J.B.; Britton, J.; Tattersfield, A.E. The effect of lifestyle on wheeze, atopy,<br>
and bronchial hyperreactivity in Asian and white children. Am. J. Respir. Crit. Care Med. 1996, 154,<br>
537–540.<br>
16. Huang, S.L.; Lin, K.C.; Pan, W.H. Dietary factors associated with physician-diagnosed asthma and<br>
allergic rhinitis in teenagers: Analyses of the first Nutrition and Health Survey in Taiwan. Clin.<br>
Exp. Allergy 2001, 31, 259–264.<br>
17. Wickens, K.; Barry, D.; Friezema, A.; Rhodius, R.; Bone, N.; Purdie, G.; Crane, J. Fast foods—<br>
Are they a risk factor for asthma? Allergy 2005, 60, 1537–1541.<br>
18. Hijazi, N.; Abalkhail, B.; Seaton, A. Diet and childhood asthma in a society in transition: A study<br>
in urban and rural Saudi Arabia. Thorax 2000, 55, 775–779.<br>
19. Varraso, R.; Kauffmann, F.; Leynaert, B.; Le Moual, N.; Boutron-Ruault, M.C.; Clavel-Chapelon,<br>
F.; Romieu, I. Dietary patterns and asthma in the E3N study. Eur. Respir. J. 2009, 33, 33–41.<br>
20. Wood, L.G.; Garg, M.L.; Gibson, P.G. A high-fat challenge increases airway inflammation and<br>
impairs bronchodilator recovery in asthma. J. Allergy Clin. Immunol. 2011, 127, 1133–1140.<br>
21. Netting, M.J.; Middleton, P.F.; Makrides, M. Does maternal diet during pregnancy and lactation<br>
affect outcomes in offspring? A systematic review of food-based approaches. Nutrition 2014, 30,<br>
1225–1241.<br>
22. Grieger, J.; Wood, L.; Clifton, V. Improving asthma during pregnancy with dietary antioxidants:<br>
The current evidence. Nutrients 2013, 5, 3212–3234.<br>
23. Butland, B.K.; Fehily, A.M.; Elwood, P.C. Diet, lung function, and lung function decline in<br>
a cohort of 2512 middle aged men. Thorax 2000, 55, 102–108.<br>
24. Carey, I.M.; Strachan, D.P.; Cook, D.G. Effects of changes in fresh fruit consumption on<br>
ventilatory function in healthy British adults. Am. J. Respir. Crit. Care Med. 1998, 158, 728–733.<br>
25. Wood, L.G.; Garg, M.L.; Smart, J.M.; Scott, H.A.; Barker, D.; Gibson, P.G. Manipulating<br>
antioxidant intake in asthma: A randomized controlled trial. Am. J. Clin. Nutr. 2012, 96, 534–543.<br>
26. Seyedrezazadeh, E.; Moghaddam, M.P.; Ansarin, K.; Vafa, M.R.; Sharma, S.; Kolahdooz, F. Fruit<br>
and vegetable intake and risk of wheezing and asthma: A systematic review and meta-analysis. Nutr.<br>
Rev. 2014, 72, 411–428.<br>
27. Erkkola, M.; Nwaru, B.I.; Kaila, M.; Kronberg-Kippilä, C.; Ilonen, J.; Simell, O.; Veijola, R.;<br>
Knip, M.; Virtanen, S.M. Risk of asthma and allergic outcomes in the offspring in relation to<br>
maternal food consumption during pregnancy: A Finnish birth cohort study. Pediatr. Allergy<br>
Immunol. 2012, 23, 186–194.<br>
28. Fitzsimon, N.; Fallon, U.; O’Mahony, D.; Loftus, B.G.; Bury, G.; Murphy, A.W.; Kelleher, C.C.;<br>
Lifeways Cross Generation Cohort Study Steering Group. Mother’s dietary patterns during<br>
pregnancy and risk of asthma symptoms in children at 3 years. Ir. Med. J. 2007, 100, 27–32.<br>
29. Baldrick, F.R.; Elborn, J.S.; Woodside, J.V.; Treacy, K.; Bradley, J.M.; Patterson, C.C.;<br>
Schock, B.C.; Ennis, M.; Young, I.S.; McKinley, M.C. Effect of fruit and vegetable intake on<br>
oxidative stress and inflammation in COPD: A randomised controlled trial. Eur. Respir. J. 2012, 39,<br>
1377–1384.<br>
30. Keranis, E.; Makris, D.; Rodopoulou, P.; Martinou, H.; Papamakarios, G.; Daniil, Z.; Zintzaras, E.;<br>
Gourgoulianis, K.I. Impact of dietary shift to higher-antioxidant foods in COPD: A randomised<br>
trial. Eur. Respir. J. 2010, 36, 774–780.<br>
31. Thies, F.; Miles, E.A.; Nebe-von-Caron, G.; Powell, J.R.; Hurst, T.L.; Newsholme, E.A.;<br>
Calder, P.C. Influence of dietary supplementation with long-chain n-3 or n-6 polyunsaturated fatty<br>
acids on blood inflammatory cell populations and functions and on plasma soluble adhesion<br>
molecules in healthy adults. Lipids 2001, 36, 1183–1193.<br>
32. Kelley, D.S.; Taylor, P.C.; Nelson, G.J.; Schmidt, P.C.; Ferretti, A.; Erickson, K.L.; Yu, R.;<br>
Chandra, R.K.; Mackey, B.E. Docosahexaenoic acid ingestion inhibits natural killer cell activity<br>
and production of inflammatory mediators in young healthy men. Lipids 1999, 34, 317–324.<br>
33. Lo, C.J.; Chiu, K.C.; Fu, M.; Chu, A.; Helton, S. Fish oil modulates macrophage P44/P42 mitogenactivated<br>
protein kinase activity induced by lipopolysaccharide. J. Parenter. Enter. Nutr. 2000, 24,<br>
159–163.<br>
34. Calder, P.C. n-3 Polyunsaturated fatty acids, inflammation, and inflammatory diseases. Am. J. Clin.<br>
Nutr. 2006, 83, 1505S–1519S.<br>
35. Peat, J.K.; Salome, C.M.; Woolcock, A.J. Factors associated with bronchial hyperresponsiveness<br>
in Australian adults and children. Eur. Respir. J. 1992, 5, 921–929.<br>
36. Tabak, C.; Wijga, A.H.; de Meer, G.; Janssen, N.A.; Brunekreef, B.; Smit, H.A. Diet and asthma<br>
in Dutch school children (ISAAC-2). Thorax 2006, 61, 1048–1053.<br>
37. Takemura, Y.; Sakurai, Y.; Honjo, S.; Tokimatsu, A.; Tokimatsu, A.; Gibo, M.; Hara, T.; Kusakari,<br>
A.; Kugai, N. The relationship between fish intake and the prevalence of asthma: The Tokorozawa<br>
childhood asthma and pollinosis study. Prev. Med. 2002, 34, 221–225.<br>
38. De Luis, D.A.; Armentia, A.; Aller, R.; Asensio, A.; Sedano, E.; Izaola, O.; Cuellar, L. Dietary<br>
intake in patients with asthma: A case control study. Nutrition 2005, 21, 320–324.<br>
39. Laerum, B.N.; Wentzel-Larsen, T.; Gulsvik, A.; Omenaas, E.; Gislason, T.; Janson, C.; Svanes, C.<br>
Relationship of fish and cod oil intake with adult asthma. Clin. Exp. Allergy 2007, 37, 1616–1623.<br>
40. McKeever, T.M.; Lewis, S.A.; Cassano, P.A.; Ocke, M.; Burney, P.; Britton, J.; Smit, H.A.<br>
The relation between dietary intake of individual fatty acids, FEV1 and respiratory disease in Dutch<br>
adults. Thorax 2008, 63, 208–214.<br>
41. Salam, M.T.; Li, Y.F.; Langholz, B.; Gilliland, F.D. Maternal fish consumption during pregnancy<br>
and risk of early childhood asthma. J. Asthma 2005, 42, 513–518.<br>
42. Klemens, C.M.; Berman, D.R.; Mozurkewich, E.L. The effect of perinatal omega-3 fatty acid<br>
supplementation on inflammatory markers and allergic diseases: A systematic review. BJOG 2011,<br>
118, 916–925.<br>
43. Thien, F.C.K.; Woods, R.; De Luca, S.; Abramson, M.J. Dietary marine fatty acids (fish oil) for<br>
asthma in adults and children (Cochrane Review). In The Cochrane Library; John Wiley & Sons,<br>
Ltd.: Chichester, UK, 2002 (updated 2010).<br>
44. Shahar, E.; Boland, L.L.; Folsom, A.R.; Tockman, M.S.; McGovern, P.G.; Eckfeldt, J.H.<br>
Docosahexaenoic acid and smoking-related chronic obstructive pulmonary disease. Am. J. Respir.<br>
Crit. Care Med. 1999, 159, 1780–1785.<br>
45. De Batlle, J.; Sauleda, J.; Balcells, E.; Gómez, F.P.; Méndez, M.; Rodriguez, E.; Barreiro, E.;<br>
Ferrer, J.J.; Romieu, I.; Gea, J.; et al. Association between ?3 and ?6 fatty acid intakes and serum<br>
inflammatory markers in COPD. J. Nutr. Biochem. 2012, 23, 817–821.<br>
46. Broekhuizen, R.; Wouters, E.F.; Creutzberg, E.C.; Weling-Scheepers, C.A.; Schols, A.M.<br>
Polyunsaturated fatty acids improve exercise capacity in chronic obstructive pulmonary disease.<br>
Thorax 2005, 60, 376–382.<br>
47. Fulton, A.S.; Hill, A.M.; Williams, M.T.; Howe, P.R.; Frith, P.A.; Wood, L.G.; Garg, M.L.;<br>
Coates, A.M. Feasibility of omega-3 fatty acid supplementation as an adjunct therapy for people<br>
with chronic obstructive pulmonary disease: Study protocol for a randomized controlled trial.<br>
Trials 2013, 14, 107.<br>
48. Texas A&M University, USA. Eicosapentaenoic Acid and Protein Modulation to Induce Anabolism<br>
in Chronic Obstructive Pulmonary Disease (COPD): Aim 2 NLM Identifier: NCT01624792.<br>
Available online: clinicaltrials.gov/ct2/show/NCT01624792 (accessed on 29 September 2014).<br>
49. National Institute of Environmental Health Sciences; Columbia University, USA. The Chronic<br>
Obstructive Pulmonary Disease Fish Oil Pilot Trial (COD-Fish). Available online:<br>
clinicaltrials.gov/show/NCT00835289 (accessed on 29 September 2014).<br>
50. Wood, L.G.; Gibson, P.G.; Garg, M.L. Biomarkers of lipid peroxidation, airway inflammation and<br>
asthma. Eur. Respir. J. 2003, 21, 177–186.<br>
51. Kelly, F.J. Vitamins and respiratory disease: Antioxidant micronutrients in pulmonary health and<br>
disease. Proc. Nutr. Soc. 2005, 64, 510–526.<br>
52. Rahman, I. Oxidative stress, chromatin remodeling and gene transcription in inflammation and<br>
chronic lung diseases. J. Biochem. Mol. Biol. 2003, 36, 95–109.<br>
53. Ochs-Balcom, H.M.; Grant, B.J.; Muti, P.; Sempos, C.T.; Freudenheim, J.L.; Browne, R.W.;<br>
McCann, S.E.; Trevisan, M.; Cassano, P.A.; Iacoviello, L.; et al. Antioxidants, oxidative stress,<br>
and pulmonary function in individuals diagnosed with asthma or COPD. Eur. J. Clin. Nutr. 2006, 60,<br>
991–999.<br>
54. Wood, L.G.; Garg, M.L.; Powell, H.; Gibson, P.G. Lycopene-rich treatments modify<br>
noneosinophilic airway inflammation in asthma: Proof of concept. Free Radic. Res. 2008, 42,<br>
94–102.<br>
55. Clifton, V.L.; Vanderlelie, J.; Perkins, A.V. Increased anti-oxidant enzyme activity and biological<br>
oxidation in placentae of pregnancies complicated by maternal asthma. Placenta 2005, 26, 773–779.<br>
56. McLernon, P.C.; Wood, L.G.; Murphy, V.E.; Hodyl, N.A.; Clifton, V.L. Circulating antioxidant<br>
profile of pregnant women with asthma. Clin. Nutr. 2012, 31, 99–107.<br>
57. Miyake, Y.; Sasaki, S.; Tanaka, K.; Hirota, Y. Dairy food, calcium and vitamin D intake in<br>
pregnancy, and wheeze and eczema in infants. Eur. Respir. J. 2010, 35, 1228–1234.<br>
58. Kumar, R. Prenatal factors and the development of asthma. Curr. Opin. Pediatr. 2008, 20,<br>
682–687.<br>
59. Bowie, A.G.; O’Neill, L.A. Vitamin C inhibits NF-kappa B activation by TNF via the activation<br>
of p38 mitogen-activated protein kinase. J. Immunol. 2000, 165, 7180–7188.<br>
60. Jeong, Y.-J.; Kim, J.-H.; Kang, J.S.; Lee, W.J.; Hwang, Y. Mega-dose vitamin C attenuated lung<br>
inflammation in mouse asthma model. Anat. Cell Biol. 2010, 43, 294–302.<br>
61. Chang, H.-H.; Chen, C.-S.; Lin, J.-Y. High Dose Vitamin C Supplementation Increases the<br>
Th1/Th2 Cytokine Secretion Ratio, but Decreases Eosinophilic Infiltration in Bronchoalveolar<br>
Lavage Fluid of Ovalbumin-Sensitized and Challenged Mice. J. Agric. Food Chem. 2009, 57,<br>
10471–10476.<br>
62. Forastiere, F.; Pistelli, R.; Sestini, P.; Fortes, C.; Renzoni, E.; Rusconi, F.; Dell’Orco, V.; Ciccone,<br>
G.; Bisanti, L. The SIDRIA Collaborative Group, I. Consumption of fresh fruit rich in vitamin C<br>
and wheezing symptoms in children. Thorax 2000, 55, 283–288.<br>
63. Emmanouil, E.; Manios, Y.; Grammatikaki, E.; Kondaki, K.; Oikonomou, E.; Papadopoulos, N.;<br>
Vassilopoulou, E. Association of nutrient intake and wheeze or asthma in a Greek pre-school<br>
population. Pediatr. Allergy Immunol. 2010, 21, 90–95.<br>
64. Cook, D.G.; Carey, I.M.; Whincup, P.H.; Papacosta, O.; Chirico, S.; Bruckdorfer, K.R.; Walker, M.<br>
Effect of fresh fruit consumption on lung function and wheeze in children. Thorax 1997, 52,<br>
628–633.<br>
65. Schwartz, J.; Weiss, S.T. Relationship between dietary vitamin C intake and pulmonary function<br>
in the First National Health and Nutrition Examination Survey (NHANES I). Am. J. Clin. Nutr.<br>
1994, 59, 110–114.<br>
66. Shaheen, S.O.; Sterne, J.A.; Thompson, R.L.; Songhurst, C.E.; Margetts, B.M.; Burney, P.G.<br>
Dietary antioxidants and asthma in adults: Population-based case-control study. Am. J. Respir. Crit.<br>
Care Med. 2001, 164, 1823–1828.<br>
67. Koike, K.; Ishigami, A.; Sato, Y.; Hirai, T.; Yuan, Y.; Kobayashi, E.; Tobino, K.; Sato, T.; Sekiya,<br>
M.; Takahashi, K.; et al. Vitamin C Prevents Cigarette Smoke–Induced Pulmonary Emphysema in<br>
Mice and Provides Pulmonary Restoration. Am. J. Respir. Cell Mol. Biol. 2013, 50, 347–357.<br>
68. Lin, Y.C.; Wu, T.C.; Chen, P.Y.; Hsieh, L.Y.; Yeh, S.L. Comparison of plasma and intake levels<br>
of antioxidant nutrients in patients with chronic obstructive pulmonary disease and healthy people<br>
in Taiwan: A case-control study. Asia Pac. J. Clin. Nutr. 2010, 19, 393–401.<br>
69. Sargeant, L.A.; Jaeckel, A.; Wareham, N.J. Interaction of vitamin C with the relation between<br>
smoking and obstructive airways disease in EPIC Norfolk. European Prospective Investigation into<br>
Cancer and Nutrition. Eur. Respir. J. 2000, 16, 397–403.<br>
70. Brigelius-Flohe, R.; Traber, M.G. Vitamin E: Function and metabolism. FASEB J. 1999, 13,<br>
1145–1155.<br>
71. Huang, J.; May, J.M. Ascorbic acid spares ?-tocopherol and prevents lipid peroxidation in cultured<br>
H4IIE liver cells. Mol. Cell. Biochem. 2003, 247, 171–176.<br>
72. Abdala-Valencia, H.; Berdnikovs, S.; Cook-Mills, J. Vitamin E isoforms as modulators of lung<br>
inflammation. Nutrients 2013, 5, 4347–4363.<br>
73. Mabalirajan, U.; Aich, J.; Leishangthem, G.D.; Sharma, S.K.; Dinda, A.K.; Ghosh, B. Effects of<br>
vitamin E on mitochondrial dysfunction and asthma features in an experimental allergic murine<br>
model. J. Appl. Physiol. 2009, 107, 1285–1292.<br>
74. McCary, C.A.; Abdala-Valencia, H.; Berdnikovs, S.; Cook-Mills, J.M. Supplemental and highly<br>
elevated tocopherol doses differentially regulate allergic inflammation: Reversibility of ?-tocopherol<br>
and ?-tocopherol’s effects. J. Immunol. 2011, 186, 3674–3685.<br>
75. He, Y.; Franchi, L.; Nunez, G. The protein kinase PKR is critical for LPS-induced iNOS production<br>
but dispensable for inflammasome activation in macrophages. Eur. J. Immunol. 2013, 43, 1147–1152.<br>
76. Fakhrzadeh, L.; Laskin, J.D.; Laskin, D.L. Ozone-induced production of nitric oxide and TNF-?<br>
and tissue injury are dependent on NF-kappaB p50. Am. J. Physiol. Lung Cell. Mol. Physiol. 2004,<br>
287, L279–L285.<br>
77. Hernandez, M.L.; Wagner, J.G.; Kala, A.; Mills, K.; Wells, H.B.; Alexis, N.E.; Lay, J.C.; Jiang, Q.;<br>
Zhang, H.; Zhou, H.; et al. Vitamin E, ?-tocopherol, reduces airway neutrophil recruitment after<br>
inhaled endotoxin challenge in rats and in healthy volunteers. Free Radic. Biol. Med. 2013, 60,<br>
56–62.<br>
78. Dow, L.; Tracey, M.; Villar, A.; Coggon, D.; Margetts, B.M.; Campbell, M.J.; Holgate, S.T. Does<br>
dietary intake of vitamins C and E influence lung function in older people? Am. J. Respir. Crit.<br>
Care Med. 1996, 154, 1401–1404.<br>
79. Smit, H.A.; Grievink, L.; Tabak, C. Dietary influences on chronic obstructive lung disease and<br>
asthma: A review of the epidemiological evidence. Proc. Nutr. Soc. 1999, 58, 309–319.<br>
80. Tabak, C.; Smit, H.A.; Rasanen, L.; Fidanza, F.; Menotti, A.; Nissinen, A.; Feskens, E.J.; Heederik,<br>
D.; Kromhout, D. Dietary factors and pulmonary function: A cross sectional study in middle aged<br>
men from three European countries. Thorax 1999, 54, 1021–1026.<br>
81. Weiss, S.T. Diet as a risk factor for asthma. Ciba Found. Symp. 1997, 206, 244–257.<br>
82. Troisi, R.J.; Willett, W.C.; Weiss, S.T.; Trichopoulos, D.; Rosner, B.; Speizer, F.E. A prospective<br>
study of diet and adult-onset asthma. Am. J. Respir. Crit. Care Med. 1995, 151, 1401–1408.<br>
83. Devereux, G.; Seaton, A. Diet as a risk factor for atopy and asthma. J. Allergy Clin. Immonol.<br>
2005, 115, 1109–1117.<br>
84. Tug, T.; Karatas, F.; Terzi, S.M. Antioxidant vitamins (A, C and E) and malondialdehyde levels in<br>
acute exacerbation and stable periods of patients with chronic obstructive pulmonary disease. Clin.<br>
Investig. Med. 2004, 27, 123–128.<br>
85. Daga, M.K.; Chhabra, R.; Sharma, B.; Mishra, T.K. Effects of exogenous vitamin E supplementation<br>
on the levels of oxidants and antioxidants in chronic obstructive pulmonary disease. J. Biosci.<br>
2003, 28, 7–11.<br>
86. Wu, T.C.; Huang, Y.C.; Hsu, S.Y.; Wang, Y.C.; Yeh, S.L. Vitamin E and vitamin C supplementation<br>
in patients with chronic obstructive pulmonary disease. Int. J. Vitam. Nutr. Res. 2007, 77,<br>
272–279.<br>
87. Agler, A.H.; Kurth, T.; Gaziano, J.M.; Buring, J.E.; Cassano, P.A. Randomised vitamin E<br>
supplementation and risk of chronic lung disease in the Women’s Health Study. Thorax 2011, 66,<br>
320–325.<br>
88. West, C.E.; Dunstan, J.; McCarthy, S.; Metcalfe, J.; D’Vaz, N.; Meldrum, S.; Oddy, W.H.;<br>
Tulic, M.K.; Prescott, S.L. Associations between maternal antioxidant intakes in pregnancy and<br>
infant allergic outcomes. Nutrients 2012, 4, 1747–1758.<br>
89. Abdala-Valencia, H.; Berdnikovs, S.; Soveg, F.W.; Cook-Mills, J.M. ?-Tocopherol Supplementation<br>
of Allergic Female Mice Inhibits Development of CD11c+CD11b+ Dendritic Cells in Utero and<br>
Allergic Inflammation in Neonates. Am. J. Physiol. Lung Cell. Mol. Physiol. 2014, 307, L482–L496.<br>
90. Litonjua, A.A.; Rifas-Shiman, S.L.; Ly, N.P.; Tantisira, K.G.; Rich-Edwards, J.W.;<br>
Camargo, C.A., Jr.; Weiss, S.T.; Gillman, M.W.; Gold, D.R. Maternal antioxidant intake in<br>
pregnancy and wheezing illnesses in children at 2 year of age. Am. J. Clin. Nutr. 2006, 84, 903–911.<br>
91. Miyake, Y.; Sasaki, S.; Tanaka, K.; Hirota, Y. Consumption of vegetables, fruit, and antioxidants<br>
during pregnancy and wheeze and eczema in infants. Allergy 2010, 65, 758–765.<br>
92. Devereux, G.; Turner, S.W.; Craig, L.C.; McNeill, G.; Martindale, S.; Harbour, P.J.; Helms, P.J.;<br>
Seaton, A. Low maternal vitamin E intake during pregnancy is associated with asthma in 5-year-old<br>
children. Am. J. Respir. Crit. Care Med. 2006, 174, 499–507.<br>
93. Devereux, G.; Barker, R.N.; Seaton, A. Antenatal determinants of neonatal immune responses to<br>
allergens. Clin. Exp. Allergy 2002, 32, 43–50.<br>
94. Wassall, H.; Devereux, G.; Seaton, A.; Barker, R. Complex effects of vitamin E and vitamin C<br>
supplementation on in vitro neonatal mononuclear cell responses to allergens. Nutrients 2013, 5,<br>
3337–3351.<br>
95. Tanaka, T.; Takahashi, R. Flavonoids and asthma. Nutrients 2013, 5, 2128–2143.<br>
96. Manach, C.; Scalbert, A.; Morand, C.; Remesy, C.; Jimenez, L. Polyphenols: Food sources and<br>
bioavailability. Am. J. Clin. Nutr. 2004, 79, 727–747.<br>
97. Hirano, T.; Higa, S.; Arimitsu, J.; Naka, T.; Shima, Y.; Ohshima, S.; Fujimoto, M.; Yamadori, T.;<br>
Kawase, I.; Tanaka, T. Flavonoids such as luteolin, fisetin and apigenin are inhibitors of<br>
interleukin-4 and interleukin-13 production by activated human basophils. Int. Arch. Allergy<br>
Immunol. 2004, 134, 135–140.<br>
98. Kawai, M.; Hirano, T.; Higa, S.; Arimitsu, J.; Maruta, M.; Kuwahara, Y.; Ohkawara, T.; Hagihara,<br>
K.; Yamadori, T.; Shima, Y.; et al. Flavonoids and related compounds as anti-allergic substances.<br>
Allergol. Int. 2007, 56, 113–123.<br>
99. Nair, M.P.; Mahajan, S.; Reynolds, J.L.; Aalinkeel, R.; Nair, H.; Schwartz, S.A.; Kandaswami, C.<br>
The flavonoid quercetin inhibits proinflammatory cytokine (tumor necrosis factor alpha) gene<br>
expression in normal peripheral blood mononuclear cells via modulation of the NF-kappa beta<br>
system. Clin. Vaccine Immunol. 2006, 13, 319–328.<br>
100. Nair, M.P.N.; Kandaswami, C.; Mahajan, S.; Chadha, K.C.; Chawda, R.; Nair, H.; Kumar, N.;<br>
Nair, R.E.; Schwartz, S.A. The flavonoid, quercetin, differentially regulates Th-1 (IFN?) and Th-2<br>
(IL4) cytokine gene expression by normal peripheral blood mononuclear cells. Biochim. Biophys.<br>
Acta Mol. Cell Res. 2002, 1593, 29–36.<br>
101. Leemans, J.; Cambier, C.; Chandler, T.; Billen, F.; Clercx, C.; Kirschvink, N.; Gustin, P.<br>
Prophylactic effects of omega-3 polyunsaturated fatty acids and luteolin on airway<br>
hyperresponsiveness and inflammation in cats with experimentally-induced asthma. Vet. J.<br>
2010, 184, 111–114.<br>
102. Li, R.R.; Pang, L.L.; Du, Q.; Shi, Y.; Dai, W.J.; Yin, K.S. Apigenin inhibits allergen-induced<br>
airway inflammation and switches immune response in a murine model of asthma.<br>
Immunopharmacol. Immunotoxicol. 2010, 32, 364–370.<br>
103. Wu, M.Y.; Hung, S.K.; Fu, S.L. Immunosuppressive effects of fisetin in ovalbumin-induced<br>
asthma through inhibition of NF-kB activity. J. Agric. Food Chem. 2011, 59, 10496–10504.<br>
104. Rogerio, A.P.; Kanashiro, A.; Fontanari, C.; da Silva, E.V.G.; Lucisano-Valim, Y.M.; Soares, E.G.;<br>
Faccioli, L.H. Anti-inflammatory activity of quercetin and isoquercitrin in experimental murine<br>
allergic asthma. Inflamm. Res. 2007, 56, 402–408.<br>
105. Garcia, V.; Arts, I.C.; Sterne, J.A.; Thompson, R.L.; Shaheen, S.O. Dietary intake of flavonoids<br>
and asthma in adults. Eur. Respir. J. 2005, 26, 449–452.<br>
106. Belcaro, G.; Luzzi, R.; Cesinaro di Rocco, P.; Cesarone, M.R.; Dugall, M.; Feragalli, B.;<br>
Errichi, B.M.; Ippolito, E.; Grossi, M.G.; Hosoi, M.; et al. Pycnogenol improvements in asthma<br>
management. Panminerva Med. 2011, 53, 57–64.<br>
107. Willers, S.M.; Devereux, G.; Craig, L.C.A.; McNeill, G.; Wijga, A.H.; Abou El-Magd, W.; Turner,<br>
S.W.; Helms, P.J.; Seaton, A. Maternal food consumption during pregnancy and asthma,<br>
respiratory and atopic symptoms in 5-year-old children. Thorax 2007, 62, 773–779.<br>
108. Holick, M.F. Vitamin D deficiency. N. Engl. J. Med. 2007, 357, 266–281.<br>
109. Hart, P.H.; Lucas, R.M.; Walsh, J.P.; Zosky, G.R.; Whitehouse, A.J.O.; Zhu, K.; Allen, K.L.;<br>
Kusel, M.M.; Anderson, D.; Mountain, J.A. Vitamin D in Fetal Development: Findings From<br>
a Birth Cohort Study. Pediatrics 2014, doi:10.1542/peds.2014-1860.<br>
110. Hart, P.H.; Gorman, S.; Finlay-Jones, J.J. Modulation of the immune system by UV radiation:<br>
More than just the effects of vitamin D? Nat. Rev. Immunol. 2011, 11, 584–596.<br>
111. Foong, R.; Zosky, G. Vitamin D deficiency and the lung: Disease initiator or disease modifier?<br>
Nutrients 2013, 5, 2880–2900.<br>
112. Hiemstra, P.S. The role of epithelial ?-defensins and cathelicidins in host defense of the lung. Exp.<br>
Lung Res. 2007, 33, 537–542.<br>
113. Martinesi, M.; Bruni, S.; Stio, M.; Treves, C. 1,25-Dihydroxyvitamin D3 inhibits tumor necrosis<br>
factor-?-induced adhesion molecule expression in endothelial cells. Cell Biol. Int. 2006, 30,<br>
365–375.<br>
114. Song, Y.; Qi, H.; Wu, C. Effect of 1,25-(OH)2D3 (a vitamin D analogue) on passively sensitized<br>
human airway smooth muscle cells. Respirology 2007, 12, 486–494.<br>
115. Zosky, G.R.; Berry, L.J.; Elliot, J.G.; James, A.L.; Gorman, S.; Hart, P.H. Vitamin D deficiency<br>
causes deficits in lung function and alters lung structure. Am. J. Respir. Crit. Care Med. 2011, 183,<br>
1336–1343.<br>
116. Pichler, J.; Gerstmayr, M.; Szepfalusi, Z.; Urbanek, R.; Peterlik, M.; Willheim, M. 1?,25(OH)2D3<br>
inhibits not only Th1 but also Th2 differentiation in human cord blood T cells. Pediatr. Res. 2002,<br>
52, 12–18.<br>
117. Gupta, A.; Sjoukes, A.; Richards, D.; Banya, W.; Hawrylowicz, C.; Bush, A.; Saglani, S.<br>
Relationship between serum vitamin D, disease severity and airway remodeling in children with<br>
asthma. Am. J. Respir. Crit. Care Med. 2011, 184, 1342–1349.<br>
118. Brehm, J.M.; Schuemann, B.; Fuhlbrigge, A.L.; Hollis, B.W.; Strunk, R.C.; Zeiger, R.S.; Weiss,<br>
S.T.; Litonjua, A.A. Serum vitamin D levels and severe asthma exacerbations in the Childhood<br>
Asthma Management Program study. J. Allergy Clin. Immonol. 2010, 126, 52–58.<br>
119. Zosky, G.R.; Hart, P.H.; Whitehouse, A.J.; Kusel, M.M.; Ang, W.; Foong, R.E.; Chen, L.; Holt, P.G.;<br>
Sly, P.D.; Hall, G.L. Vitamin D deficiency at 16 to 20 weeks’ gestation is associated with impaired<br>
lung function and asthma at 6 years of age. Ann. Am. Thorac. Soc. 2014, 11, 571–577.<br>
120. Searing, D.A.; Zhang, Y.; Murphy, J.R.; Hauk, P.J.; Goleva, E.; Leung, D.Y. Decreased serum<br>
vitamin D levels in children with asthma are associated with increased corticosteroid use. J. Allergy<br>
Clin. Immonol. 2010, 125, 995–1000.<br>
121. Xystrakis, E.; Kusumakar, S.; Boswell, S.; Peek, E.; Urry, Z.; Richards, D.F.; Adikibi, T.;<br>
Pridgeon, C.; Dallman, M.; Loke, T.K.; et al. Reversing the defective induction of IL-10 secreting<br>
regulatory T cells in glucocorticoid-resistant asthma patients. J. Clin. Investig. 2006, 116,<br>
146–155.<br>
122. Castro, M.; King, T.S.; Kunselman, S.J.; Cabana, M.D.; Denlinger, L.; Holguin, F.; Kazani, S.D.;<br>
Moore, W.C.; Moy, J.; Sorkness, C.A.; et al. Effect of vitamin D3 on asthma treatment failures in<br>
adults with symptomatic asthma and lower vitamin D levels: The VIDA randomized clinical trial.<br>
JAMA 2014, 311, 2083–2091.<br>
123. Janssens, W.; Bouillon, R.; Claes, B.; Carremans, C.; Lehouck, A.; Buysschaert, I.; Coolen, J.;<br>
Mathieu, C.; Decramer, M.; Lambrechts, D. Vitamin D deficiency is highly prevalent in COPD<br>
and correlates with variants in the vitamin D-binding gene. Thorax 2010, 65, 215–220.<br>
124. Black, P.N.; Scragg, R. Relationship between serum 25-hydroxyvitamin D and pulmonary function<br>
survey. Chest 2005, 128, 3792–3798.<br>
125. Persson, L.J.; Aanerud, M.; Hiemstra, P.S.; Hardie, J.A.; Bakke, P.S.; Eagan, T.M. Chronic<br>
obstructive pulmonary disease is associated with low levels of vitamin D. PLoS One 2012,<br>
7, e38934.<br>
126. Uh, S.T.; Koo, S.M.; Kim, Y.K.; Kim, K.U.; Park, S.W.; Jang, A.S.; Kim, D.J.; Kim, Y.H.;<br>
Park, C.S. Inhibition of vitamin D receptor translocation by cigarette smoking extracts. Tuberc.<br>
Respir. Dis. 2012, 73, 258–265.<br>
127. Wood, A.M.; Bassford, C.; Webster, D.; Newby, P.; Rajesh, P.; Stockley, R.A.; Thickett, D.R.<br>
Vitamin D-binding protein contributes to COPD by activation of alveolar macrophages. Thorax<br>
2011, 66, 205–210.<br>
128. Sundar, I.K.; Hwang, J.W.; Wu, S.; Sun, J.; Rahman, I. Deletion of vitamin D receptor leads to<br>
premature emphysema/COPD by increased matrix metalloproteinases and lymphoid aggregates<br>
formation. Biochem. Biophys. Res. Commun. 2011, 406, 127–133.<br>
129. Lehouck, A.; Mathieu, C.; Carremans, C.; Baeke, F.; Verhaegen, J.; Van Eldere, J.; Decallonne,<br>
B.; Bouillon, R.; Decramer, M.; Janssens, W. High doses of vitamin D to reduce exacerbations in<br>
chronic obstructive pulmonary disease: A randomized trial. Ann. Intern. Med. 2012, 156, 105–114.<br>
130. Baker, J.C.; Ayres, J.G. Diet and asthma. Respir. Med. 2000, 94, 925–934.<br>
131. Pogson, Z.E.K.; Antoniak, M.D.; Pacey, S.J.; Lewis, S.A.; Britton, J.R.; Fogarty, A.W.<br>
Does a low sodium diet improve asthma control? A randomized controlled trial. Am. J. Respir.<br>
Crit. Care Med. 2008, 178, 132–138.<br>
132. Matthew, R.; Altura, B. The role of magnesium in lung diseases: Asthma, allergy, and pulmonary<br>
hypertension. Magnes. Trace Elem. 1991, 10, 220–228.<br>
133. Baker, J.; Tunnicliffe, W.; Duncanson, R.; Ayres, J. Dietary antioxidants in type 1 brittle asthma:<br>
A case control study. Thorax 1999, 54, 115–118.<br>
134. Gilliand, F.; Berhane, K.; Li, Y.; Kim, D.; Margolis, H. Dietary magnesium, potassium, sodium<br>
and childrens lung function. Am. J. Epidemiol. 2002, 155, 125–131.<br>
135. Kim, J.-H.; Ellwood, P.; Asher, M.I. Diet and asthma: Looking back, moving forward. Respir. Res.<br>
2009, 10, 49–55.<br>
136. Kadrabova, J.; Mad’aric, A.; Kovacikova, Z.; Podivínsky, F.; Ginter, E.; Gazdík, F. Selenium<br>
status is decreased in patients with intrinsic asthma. Biol. Trace Elem. Res. 1996, 52, 241–248.<br>
137. Devereux, G.; McNeill, G.; Newman, G.; Turner, S.; Craig, L.; Martindale, S.; Helms, P.; Seaton,<br>
A. Early childhood wheezing symptoms in relation to plasma selenium in pregnant mothers and<br>
neonates. Clin. Exp. Allergy 2007, 37, 1000–1008.<br>
138. Burney, P.; Potts, J.; Makowska, J.; Kowalski, M.; Phillips, J.; Gnatiuc, L.; Shaheen, S.; Joos, G.;<br>
Van Cauwenberge, P.; van Zele, T.; et al. A case control study of the relation between plasma<br>
selenium and asthma in European populations: A GAL2EN project. Allergy 2008, 63, 865–871.<br>
139. Shaheen, S.O.; Newson, R.B.; Rayman, M.P.; Wong, A.P.; Tumilty, M.K.; Phillips, J.M.;<br>
Potts, J.F.; Kelly, F.J.; White, P.T.; Burney, P.G. Randomised, double blind, placebo controlled<br>
trial of selenium supplementation in adult asthma. Thorax 2007, 62, 483–490.<br>
140. Shaheen, S.O.; Newson, R.B.; Henderson, A.J.; Emmett, P.M.; Sherriff, A.; Cooke, M.; Team, A.S.<br>
Umbilical cord trace elements and minerals and risk of early childhood wheezing and eczema. Eur.<br>
Respir. J. 2004, 24, 292–297.<br>
141. Andersson, I.; Grönberg, A.; Slinde, F.; Bosaeus, I.; Larsson, S. Vitamin and mineral status in<br>
elderly patients with chronic obstructive pulmonary disease. Clin. Respir. J. 2007, 1, 23–29.<br>
142. Periyalil, H.; Gibson, P.; Wood, L. Immunometabolism in obese asthmatics: Are we there yet?<br>
Nutrients 2013, 5, 3506–3530.<br>
143. Mathis, D.; Shoelson, S.E. Immunometabolism: An emerging frontier. Nat. Rev. Immunol.<br>
2011, 11, 81.<br>
144. Medzhitov, R. Origin and physiological roles of inflammation. Nature 2008, 454, 428–435.<br>
145. Berthon, B.S.; Macdonald-Wicks, L.K.; Gibson, P.G.; Wood, L.G. Investigation of the association<br>
between dietary intake, disease severity and airway inflammation in asthma. Respirology 2013, 18,<br>
447–454.<br>
146. Procaccini, C.; Jirillo, E.; Matarese, G. Leptin as an immunomodulator. Mol. Aspects Med.<br>
2012, 33, 35–45.<br>
147. Caldefie-Chezet, F.; Poulin, A.; Vasson, M.P. Leptin regulates functional capacities of<br>
polymorphonuclear neutrophils. Free Radic. Res. 2003, 37, 809–814.<br>
148. Zarkesh-Esfahani, H.; Pockley, A.G.; Wu, Z.; Hellewell, P.G.; Weetman, A.P.; Ross, R.J.<br>
Leptin indirectly activates human neutrophils via induction of TNF-alpha. J. Immunol. 2004, 172,<br>
1809–1814.<br>
149. Lugogo, N.L.; Hollingsworth, J.W.; Howell, D.L.; Que, L.G.; Francisco, D.; Church, T.D.;<br>
Potts-Kant, E.N.; Ingram, J.L.; Wang, Y.; Jung, S.H.; et al. Alveolar macrophages from<br>
overweight/obese subjects with asthma demonstrate a proinflammatory phenotype. Am. J. Respir.<br>
Crit. Care Med. 2012, 186, 404–411.<br>
150. Shore, S.A.; Terry, R.D.; Flynt, L.; Xu, A.; Hug, C. Adiponectin attenuates allergen-induced<br>
airway inflammation and hyperresponsiveness in mice. J. Allergy Clin. Immonol. 2006, 118,<br>
389–395.<br>
151. Wood, L.G.; Gibson, P.G. Adiponectin: The link between obesity and asthma in women? Am. J.<br>
Respir. Crit. Care Med. 2012, 186, 1–2.<br>
152. Wood, L.G.; Baines, K.J.; Fu, J.; Scott, H.A.; Gibson, P.G. The neutrophilic inflammatory<br>
phenotype is associated with systemic inflammation in asthma. Chest 2012, 142, 86–93.<br>
153. Scott, H.A.; Gibson, P.G.; Garg, M.L.; Wood, L.G. Airway inflammation is augmented by obesity<br>
and fatty acids in asthma. Eur. Respir. J. 2011, 38, 594–602.<br>
154. Telenga, E.D.; Tideman, S.W.; Kerstjens, H.A.; Hacken, N.H.; Timens, W.; Postma, D.S.;<br>
van den Berge, M. Obesity in asthma: More neutrophilic inflammation as a possible explanation<br>
for a reduced treatment response. Allergy 2012, 67, 1060–1068.<br>
155. Forno, E.; Young, O.M.; Kumar, R.; Simhan, H.; Celedón, J.C. Maternal Obesity in Pregnancy,<br>
Gestational Weight Gain, and Risk of Childhood Asthma. Pediatrics 2014, 134, e535–e546.<br>
156. Franssen, F.M.; O’Donnell, D.E.; Goossens, G.H.; Blaak, E.E.; Schols, A.M. Obesity and the lung:<br>
5. Obesity and COPD. Thorax 2008, 63, 1110–1117.<br>
157. Zhou, L.; Yuan, C.; Zhang, J.; Yu, R.; Huang, M.; Adcock, I.M.; Yao, X. Circulating Leptin<br>
Concentrations in Patients with Chronic Obstructive Pulmonary Disease: A Systematic Review<br>
and Meta-Analysis. Respiration 2014, 86, 512–522.<br>
158. Bianco, A.; Mazzarella, G.; Turchiarelli, V.; Nigro, E.; Corbi, G.; Scudiero, O.; Sofia, M.; Daniele,<br>
A. Adiponectin: An attractive marker for metabolic disorders in chronic obstructive pulmonary<br>
disease (COPD). Nutrients 2013, 5, 4115–4125.<br>
159. Daniele, A.; de Rosa, A.; de Cristofaro, M.; Monaco, M.L.; Masullo, M.; Porcile, C.; Capasso, M.;<br>
Tedeschi, G.; Oriani, G.; di Costanzo, A. Decreased concentration of adiponectin together with<br>
a selective reduction of its high molecular weight oligomers is involved in metabolic complications<br>
of myotonic dystrophy type 1. Eur. J. Endocrinol. 2011, 165, 969–975.<br>
160. Kadowaki, T.; Yamauchi, T. Adiponectin and adiponectin receptors. Endocr. Rev. 2005, 26,<br>
439–451.<br>
161. Nigro, E.; Scudiero, O.; Sarnataro, D.; Mazzarella, G.; Sofia, M.; Bianco, A.; Daniele, A.<br>
Adiponectin affects lung epithelial A549 cell viability counteracting TNFa and IL-1? toxicity<br>
through AdipoR1. Int. J. Biochem. Cell Biol. 2013, 45, 1145–1153.<br>
162. Gable, D.R.; Matin, J.; Whittall, R.; Cakmak, H.; Li, K.W.; Cooper, J.; Miller, G.J.; Humphries, S.E.;<br>
HIFMECH investigators. Common adiponectin gene variants show different effects on risk of<br>
cardiovascular disease and type 2 diabetes in European subjects. Ann. Hum. Genet. 2007, 71,<br>
453–466.<br>
163. Yoon, H.I.; Li, Y.; Man, S.F.; Tashkin, D.; Wise, R.A.; Connett, J.E.; Anthonisen, N.A.; Churg, A.;<br>
Wright, J.L.; Sin, D.D. The complex relationship of serum adiponectin to COPD outcomes COPD<br>
and adiponectin. Chest 2012, 142, 893–899.<br>
164. Daniele, A.; de Rosa, A.; Nigro, E.; Scudiero, O.; Capasso, M.; Masullo, M.; de Laurentiis, G.;<br>
Oriani, G.; Sofia, M.; Bianco, A. Adiponectin oligomerization state and adiponectin receptors<br>
airway expression in chronic obstructive pulmonary disease. Int. J. Biochem. Cell Biol. 2012, 44,<br>
563–569.<br>
165. Petridou, E.T.; Mitsiades, N.; Gialamas, S.; Angelopoulos, M.; Skalkidou, A.; Dessypris, N.;<br>
Hsi, A.; Lazaris, N.; Polyzos, A.; Syrigos, C.; et al. Circulating adiponectin levels and expression<br>
of adiponectin receptors in relation to lung cancer: Two case-control studies. Oncology 2007, 73,<br>
261–269.<br>
166. Ajuwon, K.M.; Spurlock, M.E. Adiponectin inhibits LPS-induced NF-kappaB activation and<br>
IL-6 production and increases PPARgamma2 expression in adipocytes. Am. J. Physiol. Regul.<br>
Integr. Comp. Physiol. 2005, 288, R1220–R1225.<br>
167. Cheng, X.; Folco, E.J.; Shimizu, K.; Libby, P. Adiponectin induces pro-inflammatory programs in<br>
human macrophages and CD4+ T cells. J. Biol. Chem. 2012, 287, 36896–36904.<br>
168. Miller, M.; Pham, A.; Cho, J.Y.; Rosenthal, P.; Broide, D.H. Adiponectin-deficient mice are<br>
protected against tobacco-induced inflammation and increased emphysema. Am. J. Physiol. Lung<br>
Cell. Mol. Physiol. 2010, 299, L834–L842.<br>
169. Furukawa, T.; Hasegawa, T.; Suzuki, K.; Koya, T.; Sakagami, T.; Youkou, A.; Kagamu, H.;<br>
Arakawa, M.; Gejyo, F.; Narita, I.; et al. Influence of underweight on asthma control. Allergol. Int.<br>
2012, 61, 489–496.<br>
170. Harding, R.; Maritz, G. Maternal and fetal origins of lung disease in adulthood. Semin. Fetal.<br>
Neonatal Med. 2012, 17, 67–72.<br>
171. Itoh, M.; Tsuji, T.; Nemoto, K.; Nakamura, H.; Aoshiba, K. Undernutrition in patients with COPD<br>
and its treatment. Nutrients 2013, 5, 1316–1335.<br>
172. Hallin, R.; Koivisto-Hursti, U.K.; Lindberg, E.; Janson, C. Nutritional status, dietary energy intake<br>
and the risk of exacerbations in patients with chronic obstructive pulmonary disease (COPD).<br>
Respir. Med. 2006, 100, 561–567.<br>
173. Grönberg, A.M.; Slinde, F.; Engström, C.P.; Hulthén, L.; Larsson, S. Dietary problems in patients<br>
with severe chronic obstructive disease. J. Hum. Nutr. Diet. 2005, 18, 445–452.<br>
174. Wilson, D.O.; Donahoe, M.; Rogers, R.M.; Pennock, B.E. Metabolic rate and weight loss in<br>
chronic obstructive lung disease. J. Parenter. Enter. Nutr. 1990, 14, 7–11.<br>
175. Gan, W.Q.; Man, S.F.; Senthilselvan, A.; Sin, D.D. Association between chronic obstructive<br>
pulmonary disease and systemic inflammation: A systematic review and a meta-analysis. Thorax<br>
2004, 59, 574–580.<br>
176. Orlander, J.; Kiessling, K.H.; Larsson, L. Skeletal muscle metabolism, morphology and function<br>
in sedentary smokers and nonsmokers. Acta Physiol. Scand. 1979, 107, 39–46.<br>
177. Kok, M.O.; Hoekstra, T.; Twisk, J.W.R. The Longitudinal Relation between Smoking and Muscle<br>
Strength in Healthy Adults. Eur. Addict. Res. 2012, 18, 70–75.<br>
178. Gosker, H.R.; Langen, R.C.J.; Bracke, K.R.; Joos, G.F.; Brusselle, G.G.; Steele, C.; Ward, K.A.;<br>
Wouters, E.F.M.; Schols, A.M.W.J. Extrapulmonary Manifestations of Chronic Obstructive<br>
Pulmonary Disease in a Mouse Model of Chronic Cigarette Smoke Exposure. Am. J. Respir. Cell<br>
Mol. Biol. 2009, 40, 710–716.<br>
179. Nakatani, T.; Nakashima, T.; Kita, T.; Ishihara, A. Effects of exposure to cigarette smoke at<br>
different dose levels on extensor digitorum longus muscle fibres in Wistar-Kyoto and spontaneously<br>
hypertensive rats. Clin. Exp. Pharmacol. Physiol. 2003, 30, 671–677.<br>
180. Remels, A.H.; Gosker, H.R.; Langen, R.C.; Schols, A.M. The mechanisms of cachexia underlying<br>
muscle dysfunction in COPD. J. Appl. Physiol. (1985) 2013, 114, 1253–1262.<br>
181. Caron, M.A.; Debigare, R.; Dekhuijzen, P.N.; Maltais, F. Comparative assessment of the<br>
quadriceps and the diaphragm in patients with COPD. J. Appl. Physiol. (1985) 2009, 107,<br>
952–961.<br>
182. Vogiatzis, I.; Simoes, D.C.; Stratakos, G.; Kourepini, E.; Terzis, G.; Manta, P.; Athanasopoulos, D.;<br>
Roussos, C.; Wagner, P.D.; Zakynthinos, S. Effect of pulmonary rehabilitation on muscle<br>
remodelling in cachectic patients with COPD. Eur. Respir. J. 2010, 36, 301–310.<br>
183. Kythreotis, P.; Kokkini, A.; Avgeropoulou, S.; Hadjioannou, A.; Anastasakou, E.; Rasidakis, A.;<br>
Bakakos, P. Plasma leptin and insulin-like growth factor I levels during acute exacerbations of<br>
chronic obstructive pulmonary disease. BMC Pulm. Med. 2009, 9, 11.<br>
184. Barreiro, E.; Rabinovich, R.; Marin-Corral, J.; Barbera, J.A.; Gea, J.; Roca, J. Chronic endurance<br>
exercise induces quadriceps nitrosative stress in patients with severe COPD. Thorax 2009, 64,<br>
13–19.<br>
185. Plant, P.J.; Brooks, D.; Faughnan, M.; Bayley, T.; Bain, J.; Singer, L.; Correa, J.; Pearce, D.;<br>
Binnie, M.; Batt, J. Cellular markers of muscle atrophy in chronic obstructive pulmonary disease.<br>
Am. J. Respir. Cell Mol. Biol. 2010, 42, 461–471.<br>
186. Langen, R.C.; Haegens, A.; Vernooy, J.H.; Wouters, E.F.; de Winther, M.P.; Carlsen, H.; Steele, C.;<br>
Shoelson, S.E.; Schols, A.M. NF-kappaB activation is required for the transition of pulmonary<br>
inflammation to muscle atrophy. Am. J. Respir. Cell Mol. Biol. 2012, 47, 288–297.<br>
187. Sharma, R.; Anker, S.D. Cytokines, apoptosis and cachexia: The potential for TNF antagonism.<br>
Int. J. Cardiol. 2002, 85, 161–171.<br>
188. Ferreira, I.M.; Brooks, D.; White, J.; Goldstein, R. Nutritional supplementation for stable chronic<br>
obstructive pulmonary disease. Cochrane Database Syst. Rev. 2012, 12, CD000998.<br>
189. Planas, M.; Alvarez, J.; García-Peris, P.A.; de la Cuerda, C.; de Lucas, P.; Castellà, M.; Canseco, F.;<br>
Reyes, L. Nutritional support and quality of life in stable chronic obstructive pulmonary disease<br>
(COPD) patients. Clin. Nutr. 2005, 24, 433–441.<br>
190. Sugawara, K.; Takahashi, H.; Kasai, C.; Kiyokawa, N.; Watanabe, T.; Fujii, S.; Kashiwagura, T.;<br>
Honma, M.; Satake, M.; Shioya, T. Effects of nutritional supplementation combined with<br>
low-intensity exercise in malnourished patients with COPD. Respir. Med. 2010, 104, 1883–1889.<br>
191. Al-Ghimlas, F.; Todd, D.C. Creatine supplementation for patients with COPD receiving<br>
pulmonary rehabilitation: A systematic review and meta-analysis. Respirology 2010, 15, 785–795.<br>
192. Morimitsu, Y.; Nakagawa, Y.; Hayashi, K.; Fujii, H.; Kumagai, T.; Nakamura, Y.; Osawa, T.;<br>
Horio, F.; Itoh, K.; Iida, K.; et al. A sulforaphane analogue that potently activates the Nrf2-<br>
dependent detoxification pathway. J. Biol. Chem. 2002, 277, 3456–3463.<br>
193. Meja, K.K.; Rajendrasozhan, S.; Adenuga, D.; Biswas, S.K.; Sundar, I.K.; Spooner, G.;<br>
Marwick, J.A.; Chakravarty, P.; Fletcher, D.; Whittaker, P.; et al. Curcumin restores corticosteroid<br>
function in monocytes exposed to oxidants by maintaining HDAC2. Am. J. Respir. Cell Mol. Biol.<br>
2008, 39, 312–323.<br>
194. Engelen, M.P.; Rutten, E.P.; de Castro, C.L.; Wouters, E.F.; Schols, A.M.; Deutz, N.E.<br>
Supplementation of soy protein with branched-chain amino acids alters protein metabolism in<br>
healthy elderly and even more in patients with chronic obstructive pulmonary disease. Am. J. Clin.<br>
Nutr. 2007, 85, 431–439.<br>
195. Dal Negro, R.W.; Aquilani, R.; Bertacco, S.; Boschi, F.M.C.; Tognella, S. Comprehensive effects of<br>
supplemented essential amino acids in patients with severe COPD and sarcopenia. Monaldi Arch.<br>
Chest Dis. 2010, 73, 25–33.<br>
196. Varraso, R. Nutrition and asthma. Curr. Allergy Asthma Rep. 2012, 12, 201–210.
Post Disclaimer
Professional Scope of Practice *
The information herein on this entire blog site is not intended to replace a one-on-one relationship with a qualified healthcare professional or licensed physician and is not medical advice. We encourage you to make healthcare decisions based on your research and partnership with a qualified healthcare professional.
Blog Information & Scope Discussions
Our information scope is limited to Chiropractic, musculoskeletal, physical medicines, wellness, contributing etiological viscerosomatic disturbances within clinical presentations, associated somatovisceral reflex clinical dynamics, subluxation complexes, sensitive health issues, and/or functional medicine articles, topics, and discussions.
We provide and present clinical collaboration with specialists from various disciplines. Each specialist is governed by their professional scope of practice and their jurisdiction of licensure. We use functional health & wellness protocols to treat and support care for the injuries or disorders of the musculoskeletal system.
Our videos, posts, topics, subjects, and insights cover clinical matters, issues, and topics that relate to and directly or indirectly support our clinical scope of practice.*
Our office has reasonably attempted to provide supportive citations and has identified the relevant research studies or studies supporting our posts. We provide copies of supporting research studies available to regulatory boards and the public upon request.
We understand that we cover matters that require an additional explanation of how they may assist in a particular care plan or treatment protocol; therefore, to discuss the subject matter above further, please feel free to ask Dr. Alex Jimenez, DC, or contact us at 915-850-0900.
We are here to help you and your family.
Blessings
Dr. Alex Jimenez DC, MSACP, RN*, CCST, IFMCP*, CIFM*, ATN*
email: coach@elpasofunctionalmedicine.com
Licensed as a Doctor of Chiropractic (DC) in Texas & New Mexico*
Texas DC License # TX5807, New Mexico DC License # NM-DC2182
Licensed as a Registered Nurse (RN*) in Florida
Florida License RN License # RN9617241 (Control No. 3558029)
Compact Status: Multi-State License: Authorized to Practice in 40 States*
Graduate with Honors: ICHS: MSN-FNP (Family Nurse Practitioner Program)
Degree Granted. Masters in Family Practice MSN Diploma (Cum Laude)
Dr. Alex Jimenez DC, MSACP, MSN-FNP, RN* CIFM*, IFMCP*, ATN*, CCST
My Digital Business Card